Introduction
Polycyclic aromatic hydrocarbons (PAHs) are formed during natural and anthropogenic processes like volcanic eruptions, forest fires, oil refining or steel production. As a consequence, they are released to the atmosphere and, due to their hydrophobicity, they accumulate in soil and may therefore enter the food chain. Various experiments have shown that PAHs are potent mutagens and carcinogens [1]. The thermal desorption process of the removal of PAH contamination from soil can easily be monitored by thermal analysis [2]. The combination of DSC and themogravimetry documents melting and mass loss as an indication of the evaporation of volatiles. In this work, simultaneous thermal analysis (STA) was carried out using STA 449 Jupiter® instruments in order to obtain melting and boiling points as well as the vapor pressure for three exemplary PAHs, namely naphthalene, anthracene and benzo(a)pyrene.
These aromatic compounds were purchased by Alfa Aesar in high purities (naphthalene 99.6%, anthracene 99%, benzo(a) pyrene 96%).
Melting and Boiling Points
The NETZSCH model STA 449 F3 Jupiter® simultaneous thermal analyzer, equipped with a TG-DSC sample carrier type S, was used for determination of the melting and boiling points. Sealed aluminum crucibles with a pinhole of 50 μm were applied for these measurements. Thermometry of the STA instrument was based on a calibration with indium, aluminum and gold melting standards and verified with zinc to be accurately within 1 K. Nitrogen was used as a purge gas with a flow rate of 70 ml/min and heating up to 600°C was done at a constant heating rate of 10 K/min. The sample mass was approximately 20 mg.
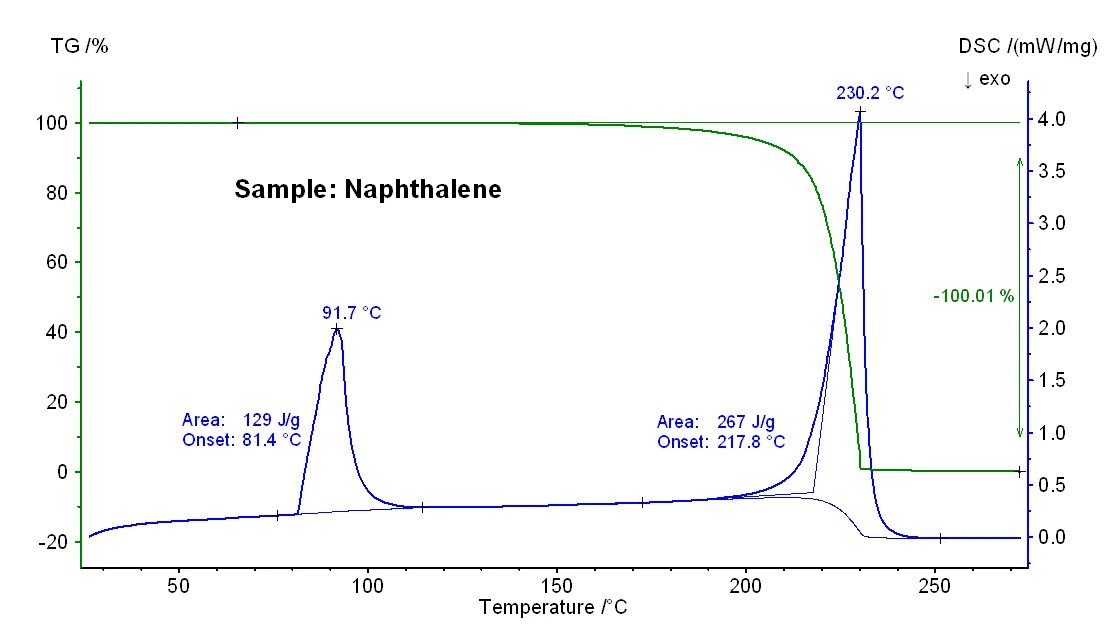
Figure 1 depicts the temperature-dependent mass changes and the DSC signal of a naphthalene sample. At an extrapolated onset temperature of 81°C, an EndothermicA sample transition or a reaction is endothermic if heat is needed for the conversion.endothermic DSC effect with an enthalpy of 129 J/g was detected which is due to melting. The extrapolated onset temperature corresponds to the Melting Temperatures and EnthalpiesThe enthalpy of fusion of a substance, also known as latent heat, is a measure of the energy input, typically heat, which is necessary to convert a substance from solid to liquid state. The melting point of a substance is the temperature at which it changes state from solid (crystalline) to liquid (isotropic melt).melting temperature, at the peak temperature of 92°C the sample is fully molten. A massloss step of 100% occurred between about 150°C and 230°C which reflects evaporation of the sample. This effect was accompanied by an EndothermicA sample transition or a reaction is endothermic if heat is needed for the conversion.endothermic DSC peak with an enthalpy of 267 J/g and an extrapolated onset temperature of 218°C. The latter reflects the boiling point of the sample.
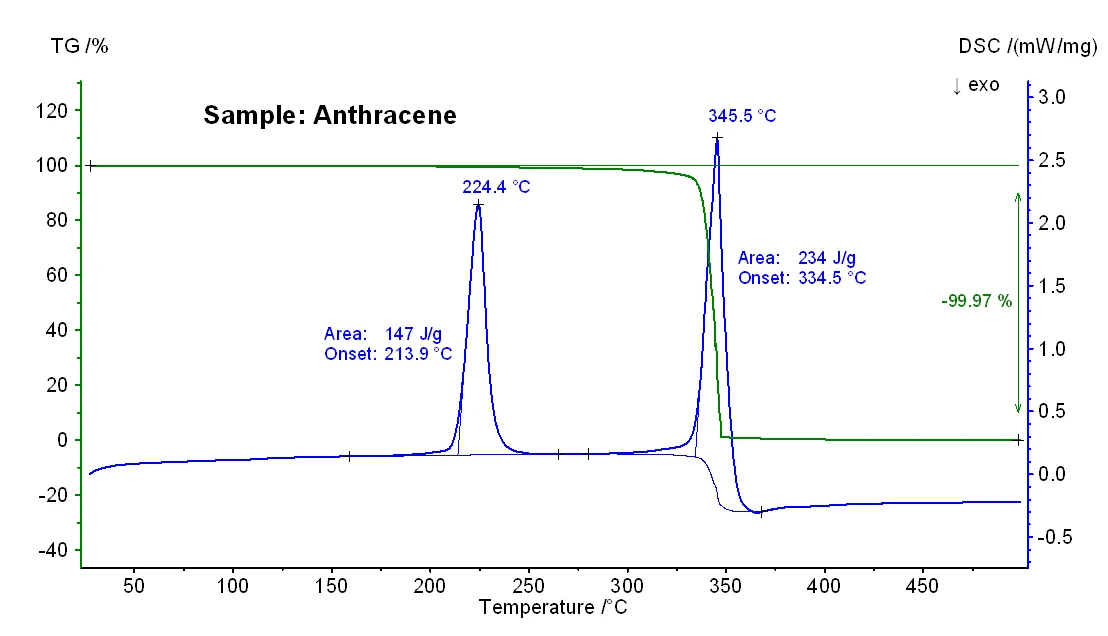
The TG-DSC results obtained for samples anthracene and benzo(a)pyrene are shown in figures 2 and 3 and the significant temperatures of melting and boiling can be seen in table 1 below. In general, it is known that particularly boiling temperatures obtained from a DSC measurement may depend on the heating rate, the initial sample mass and also on the sample preparation [3].
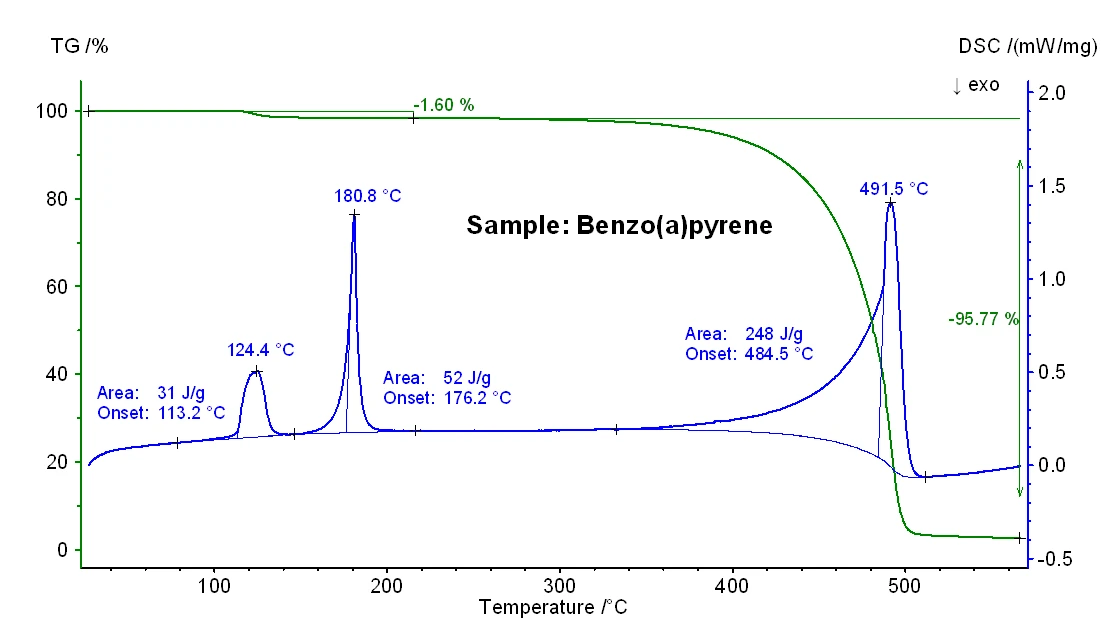
Furthermore, it should be noted that an additional mass-loss step of 1.6% as well as an EndothermicA sample transition or a reaction is endothermic if heat is needed for the conversion.endothermic effect with an enthalpy of 31 J/g were observed for the benzo(a)pyrene sample (see figure 3) which is most probably due to the release of moisture. This finding is in accordance with the lower nominal purity of this sample (see introduction).
Table 1: Comparison of nominal (in brackets, given by the supplier Alfa Aesar) and measured melting and boiling temperatures
Naphtalene | Anthrazene | Benzo(a)pyrene | |
---|---|---|---|
Melting Temperatures and EnthalpiesThe enthalpy of fusion of a substance, also known as latent heat, is a measure of the energy input, typically heat, which is necessary to convert a substance from solid to liquid state. The melting point of a substance is the temperature at which it changes state from solid (crystalline) to liquid (isotropic melt).Melting temperature | 81°C (80°C - 82°C) | 214°C (214°C - 218°C) | 176°C (177°C - 180°C) |
Boiling temperature | 218°C (218°C) | 335°C (340°C - 342°C) | 484°C (495°C) |
Vapor Pressure
The determination of the vapor pressure was realized with a STA 449 F1 Jupiter® simultaneous thermal analyzer. Instead of a standard crucible, the Knudsen cell was mounted on a TG sample carrier with thermocouple type S (see figure 4).
The vapor pressure could be obtained according to the Knudsen effusion method [4]. This method describes the evaporation of a sample material through a defined hole of the Knudsen cell into high vacuum. The STA instrument was therefore permanently evacuated during the measurement using a turbo molecular pump reaching about 10-5 mbar outside the Knudsen cell. The pressure inside the Knudsen cell is equal to the vapor pressure of the sample.
Evaporating sample material streams through the hole of the Knudsen cell leading to a mass-loss rate Δm/Δt which is the measuring quantity. The vapor pressure can be calculated according to the literature formula:

which can then be transformed into

where C is the so called Clausing correction factor [4]. This factor which depends on the ratio between the radius r and the depth l of the hole can be approximated for cylindrical holes:
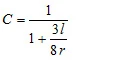
A is the area of the hole, R is the universal gas constant, T is the temperature and M is the molar mass of the sample [4]. The Knudsen effusion method is in general limited by the measurement of a finite mass-loss rate but also by the obligatory high vacuum outside the Knudsen cell. A very high mass-loss rate would lead to the break-down of the vacuum.
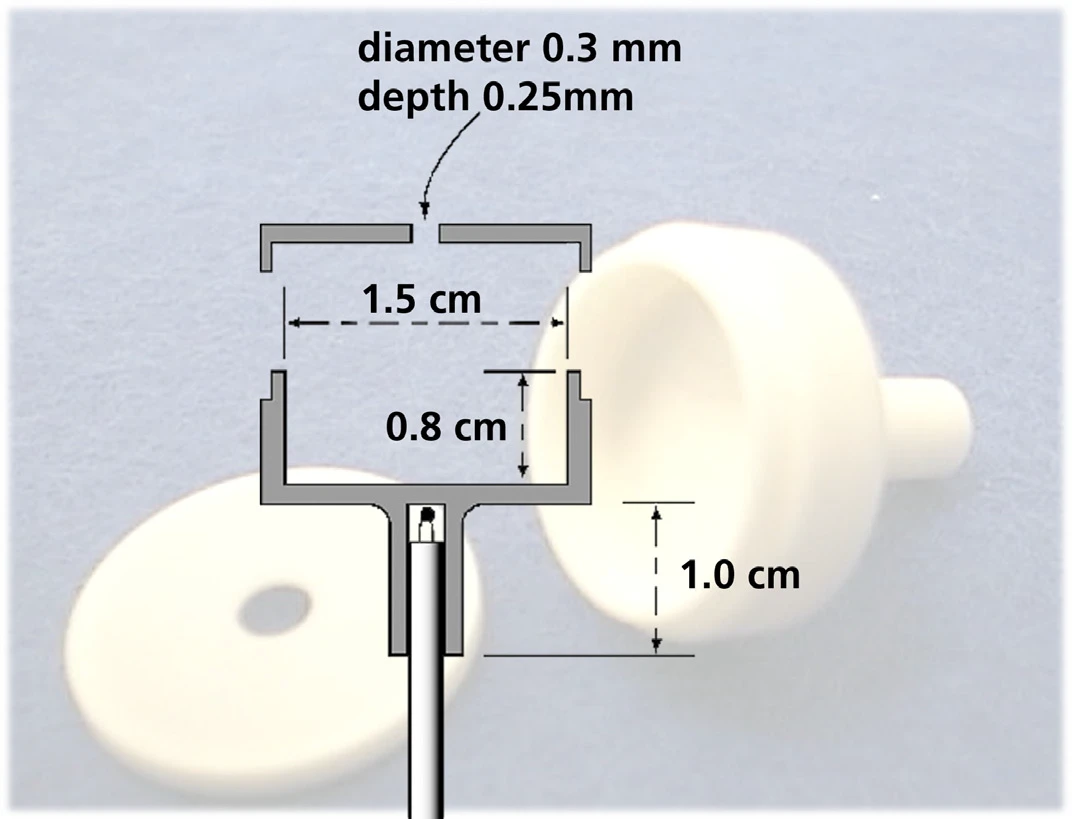
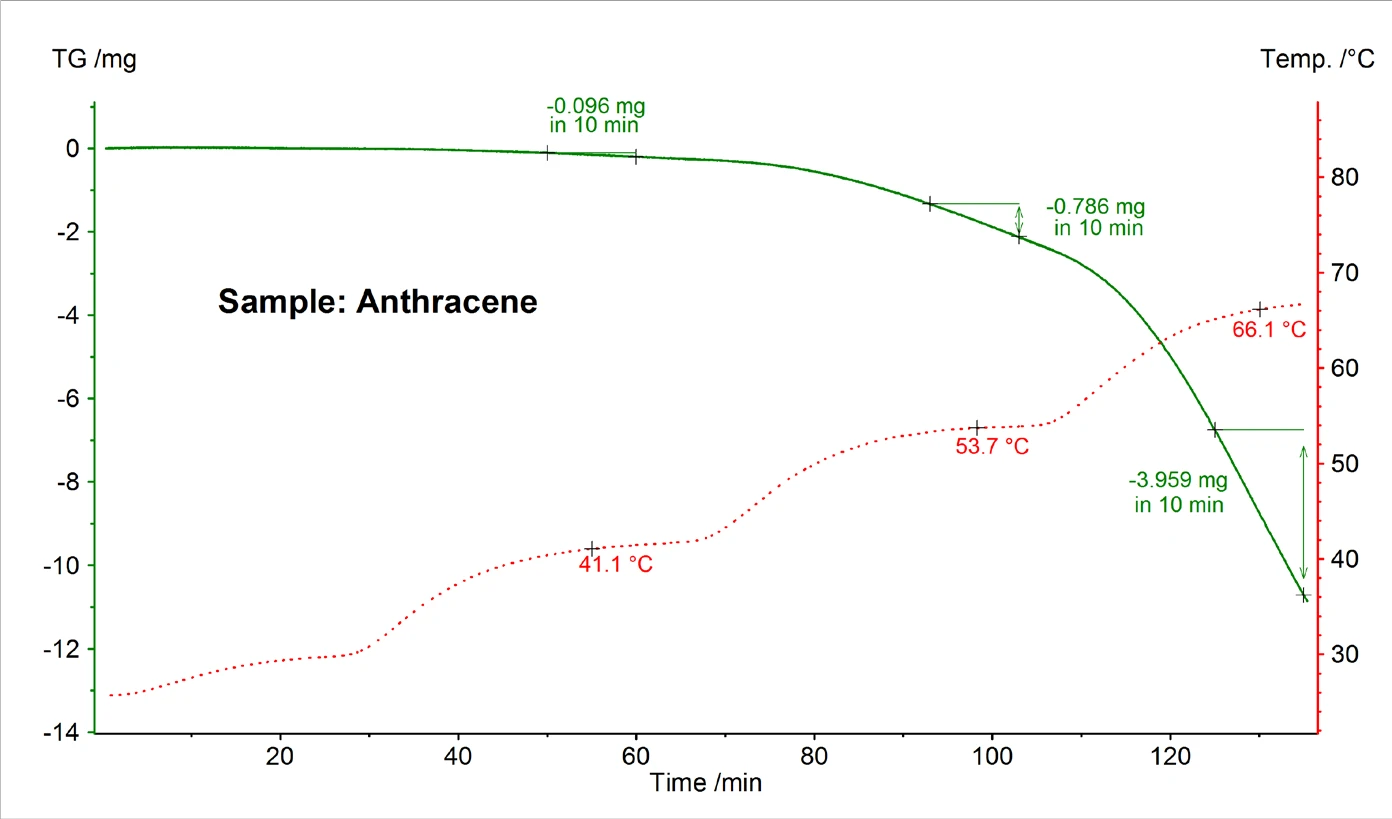
Figure 5 shows an exemplary TG measurement result for anthracene performed in high vacuum using a Knudsen cell with a hole diameter of 0.285 cm. From the mass loss rate detected at different constant temperatures, the vapor pressure was calculated using formulas (2) and (3).
The combined results obtained for anthracene, naphthalene and benzo(a)pyrene which follow the expected exponential temperature dependence can be seen in figure 6. Due to its relatively high vapor pressure, evaporation of naphthalene could only be measured close to room temperature.
The comparison with literature values [4, 5] is also shown in figure 6. A relatively large discrepancy between the measured and literature values of about one order of magnitude was found in case of benzo(a)pyrene.
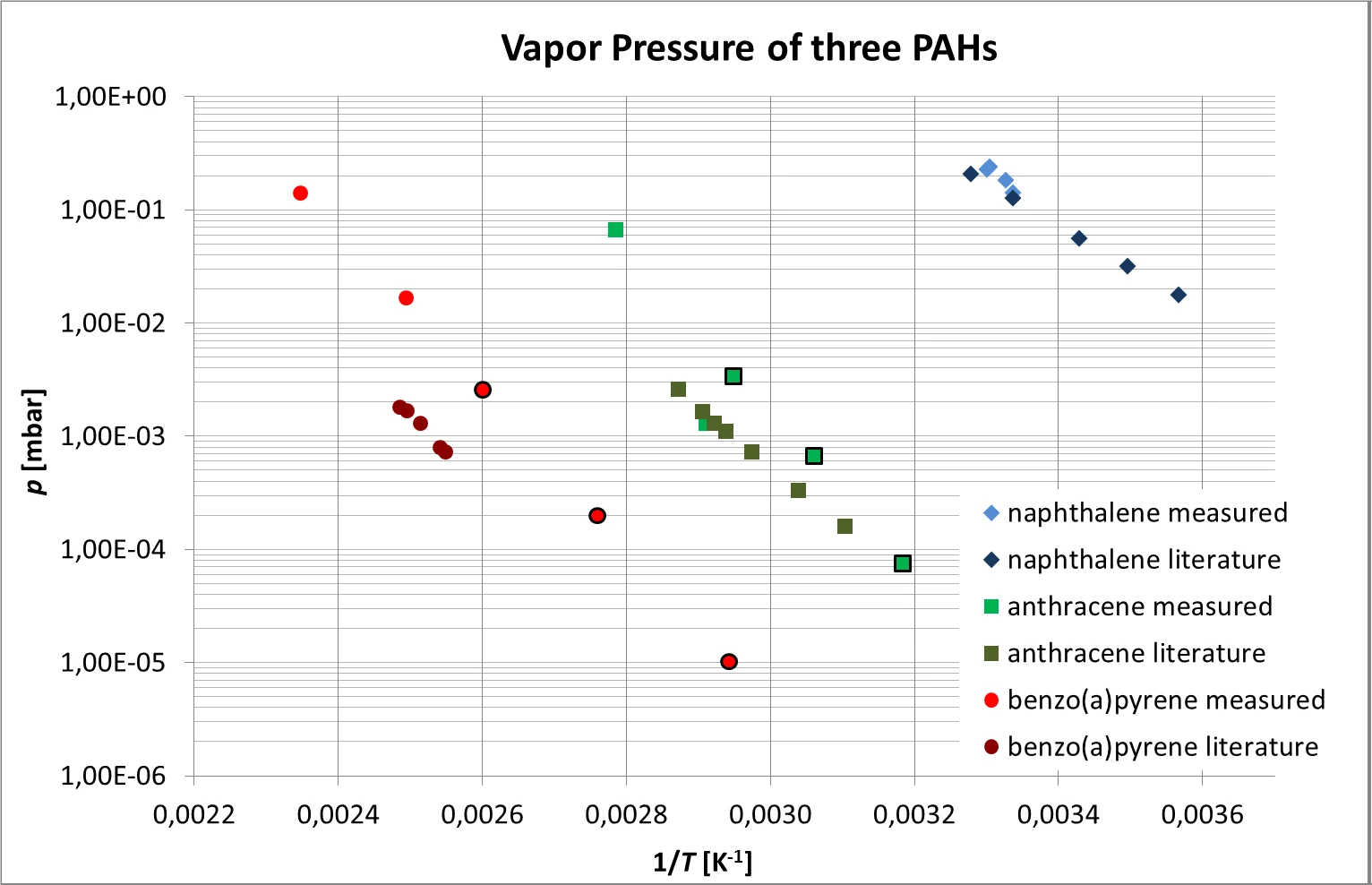
Summary
Melting Temperatures and EnthalpiesThe enthalpy of fusion of a substance, also known as latent heat, is a measure of the energy input, typically heat, which is necessary to convert a substance from solid to liquid state. The melting point of a substance is the temperature at which it changes state from solid (crystalline) to liquid (isotropic melt).Melting and boiling points of anthracene, naphthalene and benzo(a)pyrene could be identified by simultaneous thermal analysis. Vapor pressure values were furthermore determined by applying the Knudsen effusion method. All results which were obtained using the STA 449 Jupiter® instruments are in good correlation with the nominal and literature values.